In most materials, electrons move around scatter independently of one another. By contrast, electrons in quantum materials engage in highly correlated motions that resemble a complex dance. These correlations give rise to a wide range of astonishing electronic and magnetic properties, evoking profound scientific questions and challenging the field of condensed matter physics.
Research at Blusson QMI seeks to unravel and exploit the complex phenomena that emerge in novel engineered materials—not only as a result of these strong electronic correlations, but also from other sources of extraordinary behavior, such as topological states or physical structures created artificially at the atomic scale.
Our research has advanced beyond merely exploring these materials so we can now begin to rationally design materials with the ideal properties to serve as building blocks for future ultra-high-performance technologies; synthesize these materials; characterize them, developing new experimental and theoretical techniques along the way as needed; and using them to fabricate archetype devices to demonstrate their technological potential.
Research Themes
What’s in a theme? Research themes help to frame approaches, concepts, and understanding of quantum phenomena. They can help guide discussion in a complex dialogue that can have many different entry points. Because Blusson QMI evolved out of collaborations among scientists with diverse backgrounds, cultures, and expertise, in 2018, we invested in the articulation of a new Quantum Materials by Design research strategy, and identified four thematic areas.
Atomic Level Design of Quantum Materials
This area explores materials in which strong local atomic interactions play a dominant role in determining electronic behavior and physical properties. Combining multi-scale modelling and calculations with highly advanced experimental characterization techniques enables rational design of novel quantum materials.
Emergent Electronic Phenomena at Interfaces
Heterostructures of atomically smooth interfaces of quantum materials exhibit emergent properties that do not exist in bulk. This extends the capacity for the rational atomic-level design of complex structures with tunable properties, creating a bridge from materials to devices, functionalities, and applications.
Topologically Protected Quantum States
Materials with topologically protected conducting or superconducting surface states exhibit many exotic phenomena. Topological protection of quantum states offers the potential to create a new class of quantum electronic devices that can leapfrog current efforts towards realizing quantum computing.
Photonic Manipulation of Quantum States
This theme develops coherent light sources and photonic techniques to control spin, valley, and charge degrees of freedom in 2D van der Waals materials, oxide superconductors, and silicon photonic circuits, to explore unique approaches in quantum computing and discover optically driven states of matter.
Our chance to dream big

All of That Experimental Excitement in One Building
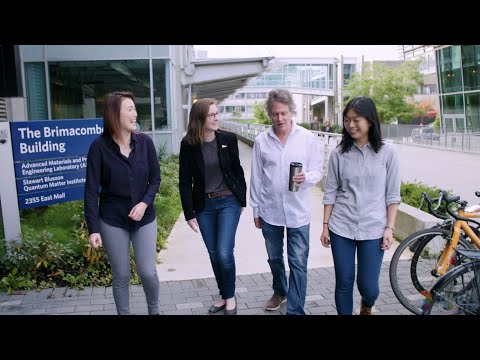