Using a novel adaptation of transport-ARPES, researchers from the Max Planck-UBC-Tokyo Centre for Quantum Materials and the Advanced Light Source at Lawrence Berkeley National Lab have experimentally unveiled the modifications of the electronic states of Ca₂RuO₄ through the current-induced insulator-to-metal transition.
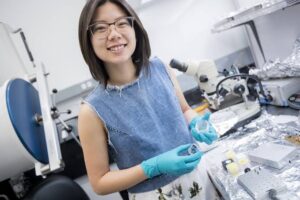
Image: Cissy Suen, a Joint PhD student with the Max Planck Institute for Solid State Research, the University of Stuttgart, and UBC’s Blusson Quantum Matter Institute (UBC Blusson QMI).
This unique transition has been observed with a simultaneous combination of transport measurements and advanced Angle-Resolved Photo Emission Spectroscopy (ARPES), offering new insights in to non-equilibrium phase transitions in correlated electron systems.
Published in Nature Physics, the study was led by Cissy Suen, a Joint PhD student with the Max Planck Institute for Solid State Research, the University of Stuttgart, and UBC’s Blusson Quantum Matter Institute (UBC Blusson QMI). Suen’s research is co-supervised by Andrea Damascelli, Scientific Director of UBC Blusson QMI in Vancouver, Canada, and Bernhard Keimer, Director of the Max Planck Institute for Solid State Research in Stuttgart, Germany.
The Physics Behind the Transition
Mott insulators, such as Ca₂RuO₄, are materials that would be metallic in the absence of interactions but behave as insulators due to strong electron-electron correlations. When current is applied, a directional dependence is imposed on the electrons, creating a new pathway toward a metallic state. Unlike conventional thermal or pressure-induced phase transitions, this current-driven transition leads to a unique electron population of the orbitals, resulting in an electronic structure distinct from that of the high-temperature metallic phase.
Probing the Transition with Transport-ARPES
To investigate the changes through this current-driven transition, researchers made significant advances in the technique of transport-ARPES. By firing high-energy photons at the material, ARPES allows for the measurement of the momentum and energy of ejected electrons, thus mapping the electronic band structure.
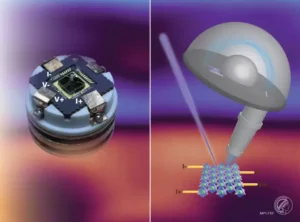
Image: Experimental configuration for Transport-ARPES.
One of the challenges with combining ARPES and transport measurements, particularly with continuous current flow, is the presence of electromagnetic fields generated by the current. These stray fields change the outgoing trajectory of the emitted photoelectrons; this has limited the use of transport-ARPES thus far to materials with clearly defined features in energy, such as the Dirac point in graphene.
By employing core-level spectroscopy, the research team successfully revealed a significant reduction in the Mott gap and modifications in the dispersion of the Ru bands. These changes indicate that the current-induced metallic phase (L*) is electronically distinct from the metallic phase induced by high temperatures (L phase). This finding points to a unique interplay between lattice structure, electronic states, and current flow, offering a deeper understanding of non-equilibrium phenomena in quantum materials.
Implications for Quantum Materials Research
The development of future technologies will rely on understanding the physics of devices in operation. As core levels can be measured for all materials, this naturally extends transport-ARPES to the study of all ARPES-suitable materials, opening the door to further study of electronic structures in devices. Furthermore, these findings present new opportunities for studying non-equilibrium states in other quantum materials, potentially revealing new quantum phases and behaviours.
By extending transport-ARPES and combining it with theoretical modelling, researchers are beginning to unravel the complex coupling between electronic, magnetic, and structural properties in quantum materials under device conditions. This work not only advances our understanding of the current-induced Mott transition, but also provides a platform for exploring physical phenomena in a broad range of correlated materials-based devices.
The Joint International PhD Program in Quantum Materials is an exciting academic program connecting UBC’s Blusson QMI and the University of Stuttgart, with participation from the Max Planck Institute for Solid State Research in Stuttgart.
The program offers unparalleled opportunities to study in the fields of quantum materials and quantum materials-based devices, and its highly collaborative nature exposes graduate student scientists to a wide variety of experimental and theoretical techniques, as well as materials systems beyond their immediate research projects, empowering students to contribute to a rapidly evolving research frontier.
This article was originally published by the Max Planck Institute for Solid State Research here.
The study published in Nature Physics is available here.